The Role of Polyphenols and Botanicals in Reprogramming Metabolism
According to the World Health Organization, one-third of all cancer deaths are preventable through an increased consumption of natural compounds able to modulate key molecular signaling cascades that ultimately inhibit cancer cell proliferation and induce apoptosis.[1],[2],[3],[4],[5],[6]
One of the many cancer targeting effects of botanical and food compounds is their ability to increase efficiency of energy metabolic pathways and potentially reprogram cancer cell metabolism. The metabolic properties of malignant cells differ significantly from those of normal cells, providing the potential to target cellular metabolism to improve the selectivity of anticancer therapeutics.[7]
Cancer Metastasis and Metabolic Reprogramming
Metastasis and metabolic reprogramming are two major hallmarks of cancer. To effectively treat cancer, both of these processes must be addressed. Metabolism is any process a cell utilizes to meet its energetic demand for biological functions. In the initiation and progression of cancer, tumor cells undergo fundamental metabolic changes to sustain their development and progression.[8]
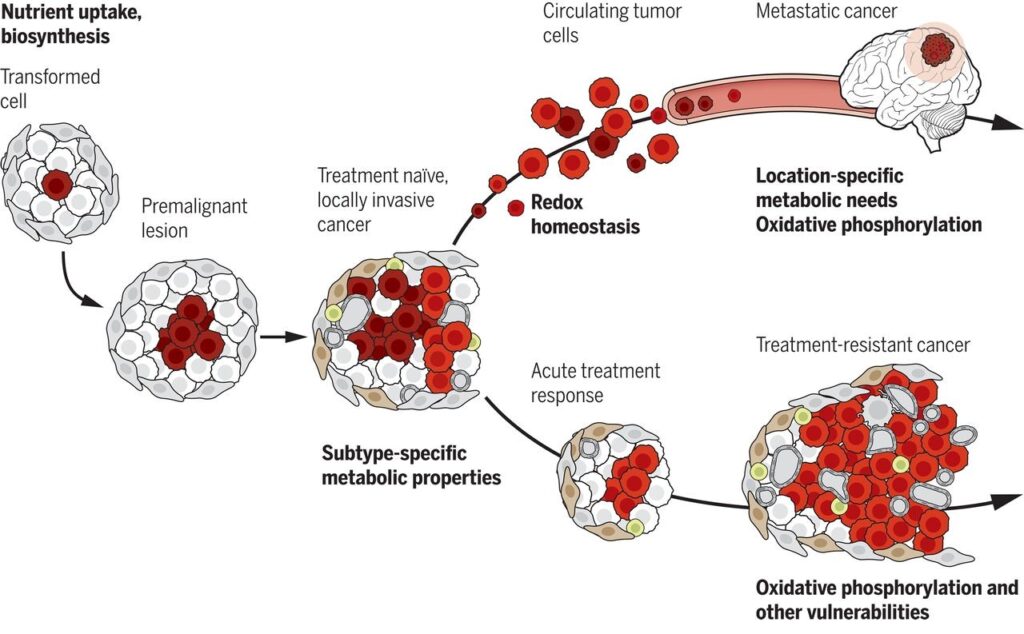
Metabolic needs and vulnerabilities evolve throughout cancer progression. Early stages of tumor growth require nutrient uptake and biosynthesis, with additional subtype-selective metabolic needs emerging in locally invasive cancers. During later stages of cancer progression, particularly metastasis and therapy resistance, tumors acquire dependence on new pathways for their growth and survival. These include potentially targetable liabilities such as dependence on mechanisms to resist oxidative stress and increased reliance on oxidative phosphorylation (OXPHOS). OXPHOS is the final stage of cellular respiration in ATP (adenosine triphosphate) production which provides most of the energy (fuel) used by the cells in our bodies for metabolism.[9]
How Cancer Cells Survive and Metastasize
Cancer cells are well documented to rewire their metabolism and energy production networks to support and enable rapid proliferation, continuous growth, survival in harsh conditions, invasion, metastasis, and resistance to cancer treatments. Common ‘mutational signatures’ (characteristic combinations of mutation types) across diverse types of cancer promote abnormal fuel utilization by the neoplastic cells themselves. This initial discovery, known as the Warburg effect, underlies the propensity of cancer cells to uptake glucose. This ultimately produces elevated amounts of lactic acid (a byproduct of anaerobic respiration) through glycolysis (the first stage of cellular respiration where ATP is produced from the conversion of glucose), as opposed to oxidative phosphorylation.[10]
Both normal cells and cancer cells metabolize nutrients, primarily glucose, to produce energy in the form of ATP. Over time, cancer cells reprogram their metabolism in order to gain access to nutrients. Sugar is no longer completely oxidized for energy production, but intermediates are increasingly used for growth and rapid cell division.[11] These changes in energy metabolism may be potential biomarkers and therapeutic targets for cancer as they frequently occur within cancer cells.[12]
Cancer cells metabolically steal nutrients, which they utilize for self-renewal and proliferation. This process also enables progression of cancer and contributes to cancer cell drug resistance. Glucose metabolism and oxidative phosphorylation, along with the metabolism of nucleotides, fatty acids, and glutamine (as well as proline, glycine, and tyrosine, specific to melanoma) all seem to have important roles based on the conditions in the tumor micro-environment. Hypoxia-inducible genes (genes that modulate oxygen homeostasis and metabolic activation in the cellular environment) and MYC target genes (a family of regulator genes and proto-oncogenes that code for transcription factors) are key players in this process.
The complex landscape of tumor metabolism in the context of the dynamic, bi-directional crosstalk with its stromal environment (connective tissue framework) is a rapidly evolving field that increasingly supports the view of cancer both as a metabolic disease and a disease of impaired cellular communication.[13]
Recent research shows that cancer cells are not solely dependent on glycolysis for their energy requirement but also derive energy from mitochondrial respiration. Invasive migratory cancer cell types and specifically cancer stem cells have been shown to display metabolic heterogeneity and prefer OXPHOS. The cellular function, fuel type and microenvironment cues, and the interplay between these plays a central regulating role in energy metabolism in cancer cells.[14],[15],[16],[17], [18] It’s important to note that cancer cells have the ability to switch between energy states such as the substrates for energy production like pyruvate or glutamine via the tricarboxylic acid cycle and OXPHOS.[19]
Glycolysis in Cancer
Cancer cells increase glucose flux to meet anabolic demands and to maintain the redox state.
Growing tumor cells can crowd out other cells and cut them off from oxygen-carrying blood vessels necessary for their survival. When this happens, as previously mentioned, some cancer cells have developed the ability to bypass the need for oxygen and instead switch to the glycolytic pathway, which they use even when oxygen is restored. Compared to normal cells, cancer cells initially prefer using glycolysis even in normal oxygen conditions. Tumor glycolysis is often called ‘aerobic glycolysis’, which is another name for the Warburg effect, to distinguish from the normal anaerobic glycolysis of healthy cells. In 1956, Otto Warburg wrote that if we know how cancer cells have “damaged respiration and excessive fermentation” (referring to the anaerobic process of glucose metabolism despite the presence of oxygen), then we understand the origin of cancer cells.[20]
Understandably, the metabolic switch from mitochondrial respiration to glycolysis during hypoxia (where oxidative phosphorylation will be inactive), as well as mitochondrial dysfunction, is critical for cancer cell growth. The increased fermentation favors the conversion of pyruvate to lactate over pyruvate metabolism in the mitochondria. Warburg proposed that the “driving force of the increased fermentation is the energy deficiency” in order to generate sufficient ATP in lieu of the defective respiration process.[21],[22]
Cancer cells preferentially express transporters and enzyme isoforms that drive glucose flux forwards.
Glycolysis produces ATP with lower efficiency, but at a faster rate than OXPHOS. This faster rate of ATP production is thought to aid the rapid proliferation of cancer cells. In addition to providing ATP, the high glycolytic rate may favor the growth of cancer cells through increasing biosynthesis of important molecules such as lipids, nucleotides, NADPH and amino acids. Other metabolic products of glycolysis, such as lactate, cause a consistent acidification of the extracellular environment and favor cancer invasion.
A number of oncogenes are known to be involved in the metabolic switch from OXPHOS to glycolysis in tumor cells, such as AMP-activated protein kinase (AMPK), hypoxia-inducible factor 1α (HIF-1α), Myc, and PI3K/Akt/mTOR. Targeting these oncogenes may selectively kill cancer cells by inhibiting the glycolytic pathway.
HIF-1α is one of the key transcription factors that plays a major role in tumor glycolysis by stimulating glucose transporters (GLUT). Glucose transporters induce the expression of glycolytic enzymes, such as hexokinase, pyruvate kinase, and lactate dehydrogenase, so that glucose is more readily taken up by the cell and used for energy.[23],[24] Glucose is taken up by cells via dedicated glucose transporters (GLUT1–4). GLUT1 and 3 appear to be the predominant glucose transporters in many types of cancer.[25]Additionally, the enzyme Hexokinase 2 (HK2), which phosphorylates glucose, is preferentially expressed by cancer cells and can be systemically deleted in mice for cancer therapy without adverse consequences.[26]
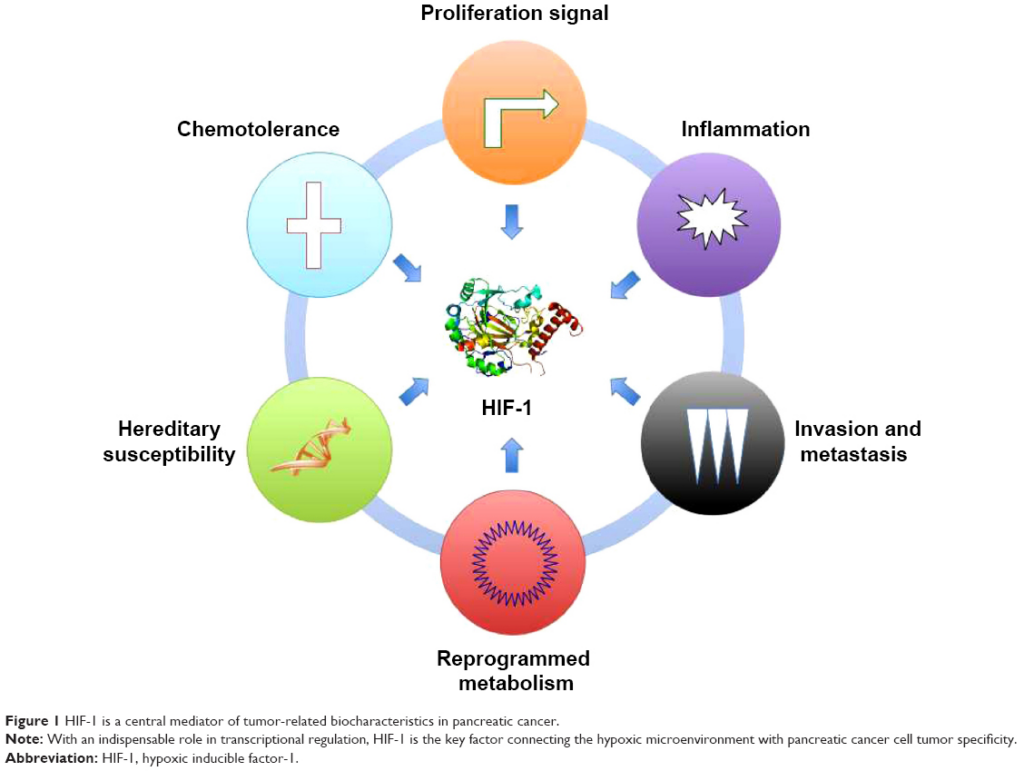
GLUT1 Expression in High-Risk Prostate Cancer: Correlation with F-FDG-PET/CT and Clinical Outcome
18F-fluorodeoxyglucose (FDG) PET/CT is a pivotal imaging modality for cancer imaging, assisting diagnosis, staging of patients with newly diagnosed malignancy, restaging following therapy and surveillance. Research indicates that tumor F-FDG-uptake is of prognostic value in high-risk and metastatic prostate cancer. Studies investigating the underlying glucose metabolism mechanisms of F-FDG-uptake on PET/CT imaging in prostate cancer provide essential data for more effective treatments for the disease.
Researchers conducted a retrospective analysis of 94 patients diagnosed with a Gleason sum ≥8 adenocarcinoma of the prostate at biopsy between July 2011 and July 2014 who underwent F-FDG-PET/CT imaging before radical prostatectomy. F-FDG-uptake in the primary lesion was measured by a blinded reader using maximum standardized uptake value (SUVmax). GLUT1, GLUT12, and HK2 expression were blindly scored after immunohistochemistry on specimens’ radical prostatectomy by three pathologists. Correlations between GLUT1, GLUT12, and HK2, and SUVmax were assessed using Spearman’s rank correlation test. Survival probabilities were based on the Kaplan–Meier method.
With a median follow-up of 4.5 years, 56% of patients had biochemical recurrence, 7% progressed to castration-resistant prostate cancer, 13% developed metastasis, and 6% died. Correlation was found between GLUT1 expression and SUVmax level. In addition, SUVmax was significantly higher in tumors with high GLUT1 expression than tumors with low GLUT1 expression. Moreover, a significant association was found between GLUT1 expression levels and SUVmax level, lymph node status (p = 0.05), volume of cancer, castration-resistant disease progression and metastasis development.
GLUT1 expression in prostate cancer tumors correlates with F-FDG-uptake and poor prognostic factors. These results suggest that this transporter is involved in the molecular mechanism of F-FDG-uptake in high-risk prostate cancer and raise interest in targeting metabolic dependencies of prostate cancer cells as a selective anticancer strategy.[27]
Glycometabolism Reprograming
Botanical compounds can help reduce HIF-1α and GLUT, as well as enhance AMPK. These strategies can help people who are suffering from cancer.
Hypoxia-inducing factors act as master regulators of stemness properties and altered metabolism of cancer and metastasis initiating cells.[28] Many herbal and dietary compounds, including cardiac glycosides, suppress the maintenance of stemness and malignancy via inhibition of HIF-1α.[29]
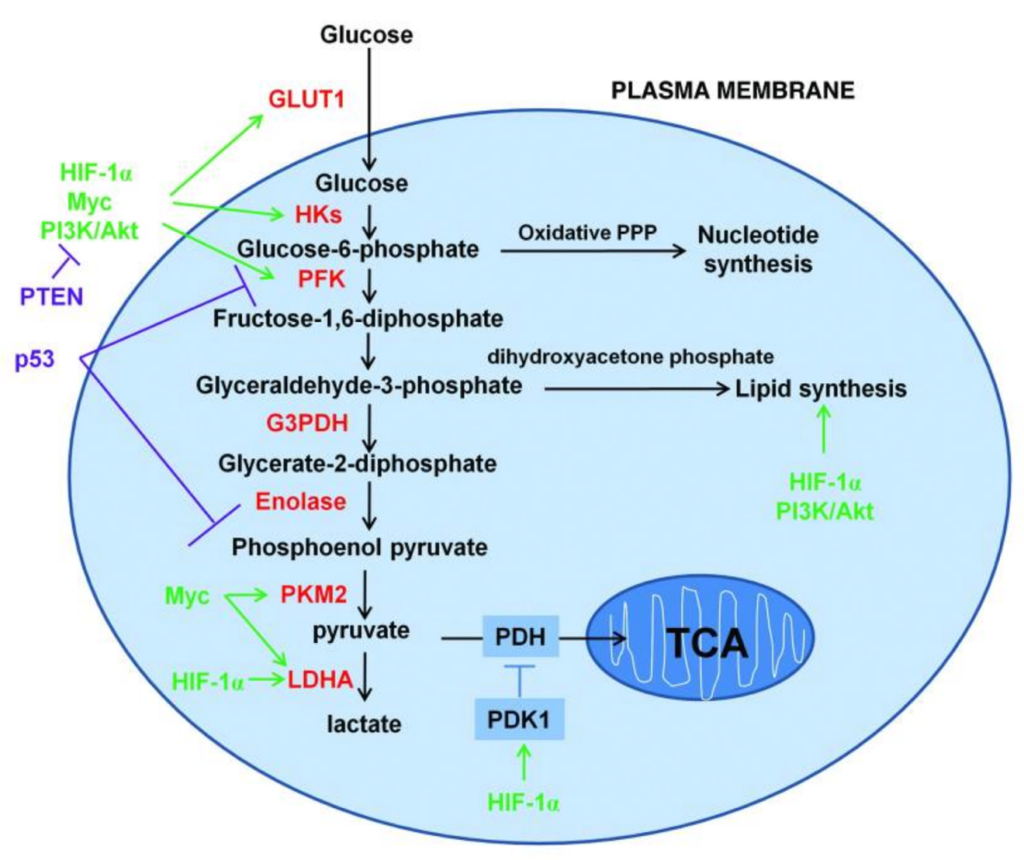
Above is a schematic representation of the glucose metabolism in cancer cells. Cancer cells increase the uptake and metabolism of glucose by regulating key transporters and enzymes (shown in red) involved in glycolytic pathways. Key oncogenic pathways are shown in green and key tumor suppressor pathways are shown in purple. The oxidative branch of pentose phosphate pathway is required in nucleotide synthesis and dihydroxyacetone phosphate pathway is critical for lipid synthesis.
Many botanical compounds act to regulate glucose transporters as well as aid in normalizing the extracellular signaling effectors. This includes upregulating AMP-activated protein kinase (AMPK), acting as an insulintrophic aid, and through peroxisome proliferator-activated receptors (PPARs) signaling.[30],[31]
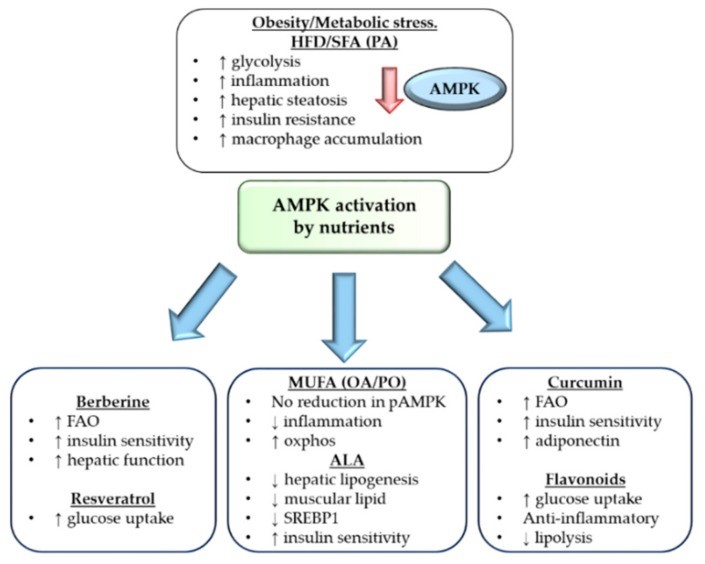
Excess nutrient consumption through high fat diet and obesity can downregulate AMPK expression and cause dysregulated metabolism, inflammation and insulin resistance. Nutrients, including monounsaturated fatty acids, α linoleic acid, berberine, resveratrol, curcumin and flavonoids can all activate AMPK and downstream positive effects in relation to improved mitochondrial metabolism, improved liver function and reduced inflammation. Therefore, modulation of AMPK through nutrient intervention can improve whole body metabolism.[32]
Ketogenesis Pathway Up-Regulates Cancer Signaling Pathway BRAF
Ketone bodies (3-hydroxy-butyrate, aceto-acetate and acetone) are naturally occurring mitochondrial fuels that are normally produced in the liver during periods of starvation. Human tumors may also share the same type of metabolic wiring as the brain. Mitochondrial dysfunction produces ketone bodies in the tumor, thus, ketone bodies behave as onco-metabolites.[33] Emerging evidence suggests these metabolites could function as signaling molecules to allow crosstalk between metabolic pathways and cell signaling networks. These findings represent a realm of crosstalk with “back and forth” signal flows between metabolic and cell signaling networks that acutely regulate cell metabolism and proliferation, which, unfortunately, are hijacked by cancer cells.
Ketogenesis mainly occurs in the mitochondria of liver cells, which normally convert acetyl-coenzyme A to ketone bodies via HMG-CoA as a result of fatty acid breakdown to generate energy when glucose levels in the blood are low. HMGCL, the gene responsible for making the enzyme HMG-CoA lyase, plays a critical role in breaking down proteins and fats from the diet. HMGCL is upregulated in human melanoma cell lines that express a specific mutation in the BRAF gene, BRAF V600E, compared to cells expressing BRAF WT (wild type) (meaning no mutation present).[34]
Why You Shouldn’t Avoid Naturally Sweet Fruits, Vegetables, Or Whole Grains
A large class of GLUT inhibitors is represented by polyphenols, a diverse family of natural compounds widely distributed in plants and in the human diet (e.g., in medicinal herbs, fruits, vegetables and beverages such as tea and wine). Although fruits contain natural sugars, they constitute a large body of diverse polyphenols, which have demonstrated an ability to suppress cancer cell glucose uptake.
Many people with cancer have the misconception that fruits, sweet vegetables, and whole grains can promote cancer growth because of the natural sugar content. This couldn’t be farther from the truth. I have written extensively on the health benefits of whole grains. When it comes to fruits, there is no research whatsoever that demonstrates whole fruits encourage cancer growth and in fact, research supports the benefits of fruits and vegetables against cancer. To suggest that naturally sweet fruits, grains, or vegetables promote cancer is theoretical and misguided. The fiber and other health-promoting components of whole fruits modulate the effects of fructose so, while the level of glucose in the blood is increased, it does not affect insulin in the same way as refined sugars and starches.
The first book I read in the mid-1970s on cancer was called My Grape Cure, written by Johanna Brandt in the 1920s. Her diet involved consuming nothing other than freshly pressed grape juice, made from purple (Concord) grapes with their skin and seeds.
Polyphenols in Grape as GLUT Inhibitors
Fresh grape juice that includes the skin and seeds contains several nutrients that have demonstrated cancer suppressing effects, including:
- ellagic acid,
- catechin,
- quercetin,
- oligomeric proanthocyanidins (OPC) or procyanidolic oligomers (PCO), originally called pycnogenol (seeds),
- resveratrol (skin coloring of purple grapes),
- pterostilbene,
- selenium,
- lycopene,
- lutein,
- laetrile (amygdalin or Vitamin B17) (seeds)
- beta-carotene,
- caffeic acid and/or ferulic acid (together they kill cancer cells), and
- gallic acid.
For example, ellagic acid, a polyphenolic compound found in pomegranates, strawberries, and walnuts, can selectively induce ROS-mediated apoptosis in cancerous B-lymphocytes of CLL patients by directly targeting mitochondria, while inhibiting ROS damage to healthy cells.[35],[36]
One of the richest sources of anthocyanin polyphenols is purple sweet potato. Research shows that purple sweet potato attenuates fat-induced mortality in fruit flies.[37]
Purple sweet potato anthocyanin (PSPA) has also been shown to have antitumor abilities. In studies, PSPA exerts antitumor effects in bladder cancer.
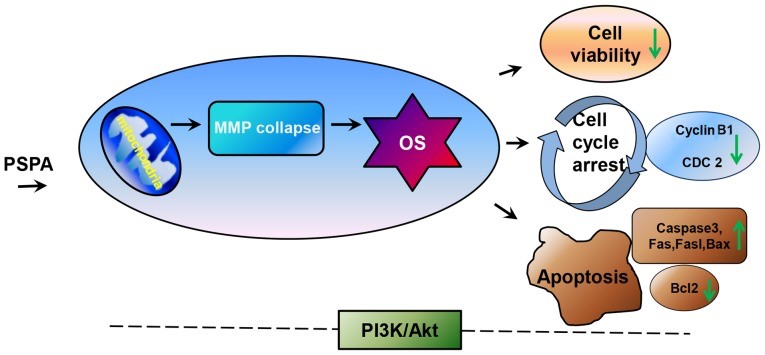
In this study, researchers speculate that mitochondria may be the first target of PSPA in cells. The dysfunction of mitochondria induces oxidative stress, thereby decreasing cell viability, inducing apoptosis and cell cycle arrest. In addition, PI3K/Akt is involved in this process.[38]
Botanicals contain bioactive compounds, such as phenolic compounds, isoprenoids, and alkaloids that are unique, due to their diverse structures and multiple targets. These ‘smarty plants’ have the ability to reprogram molecular and cell energy pathways. They normalize cell behavior and are able to regulate the behavior of both healthy cells and cancer cells—even when the actions called for are opposite (for example, blocking the uncontrolled growth of cancer cells while encouraging the growth of healthy cells).[39]
Several botanicals have been shown to interfere with the complex and interrelated biochemical reactions that result in cancer cells achieving metabolically reprogrammed dysfunctional energy homeostasis.
Metabolic reprogramming in cancer cells has four core elements:
- glucose transport,
- glycolysis,
- mitochondrial oxidative phosphorylation, and
- fatty acid synthesis.[40]
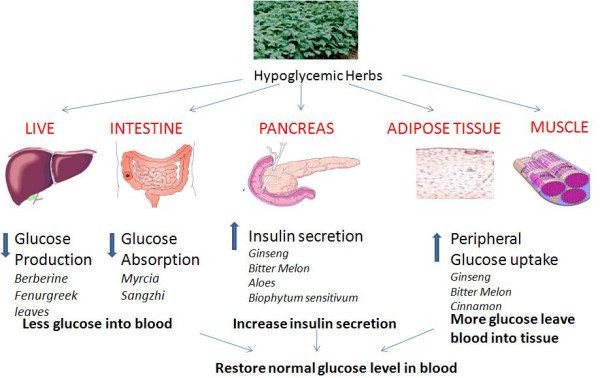
Many polyphenolic compounds exert appreciable activity on several distinct membrane transporters, including GLUTs.
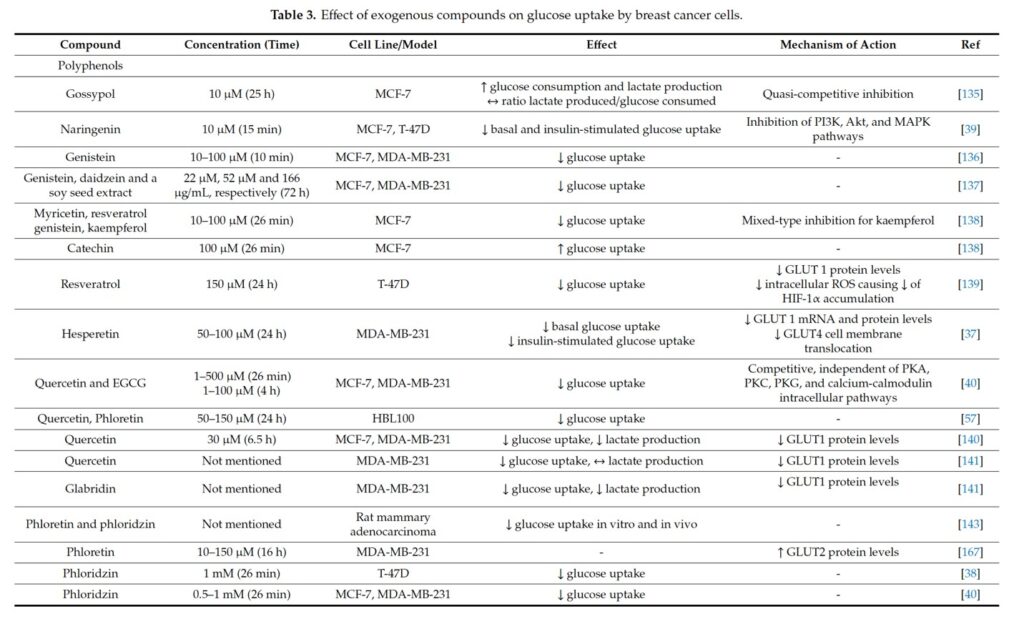
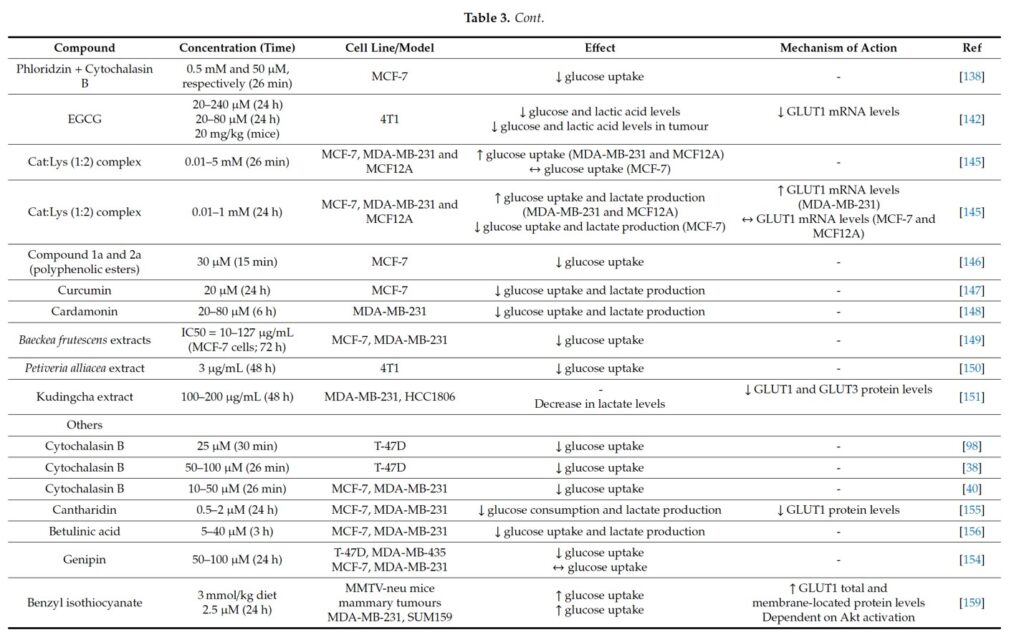
Bitter Melon Extract (BME) Suppresses Oral Cancer by Inhibiting the Key Metabolic Pathways, Glycolysis and Lipogenesis
Bitter melon (Momordica charantia), a member of the Cucurbitaceae family, is well-known for its hypoglycemic, anti-diabetic effects. Treatment with BME on oral cancer cell lines significantly reduced mRNA and protein expression levels of key glycolytic genes SLC2A1 (GLUT-1), PFKP, LDHA, PKM and PDK3. Pyruvate and lactate levels and glycolysis rate were reduced in oral cancer cells following BME treatment. In the lipogenesis pathway, a significant reduction of genes involved in fatty acid biogenesis, ACLY, ACC1 and FASN, was observed at the mRNA and protein levels following BME treatment.[43]
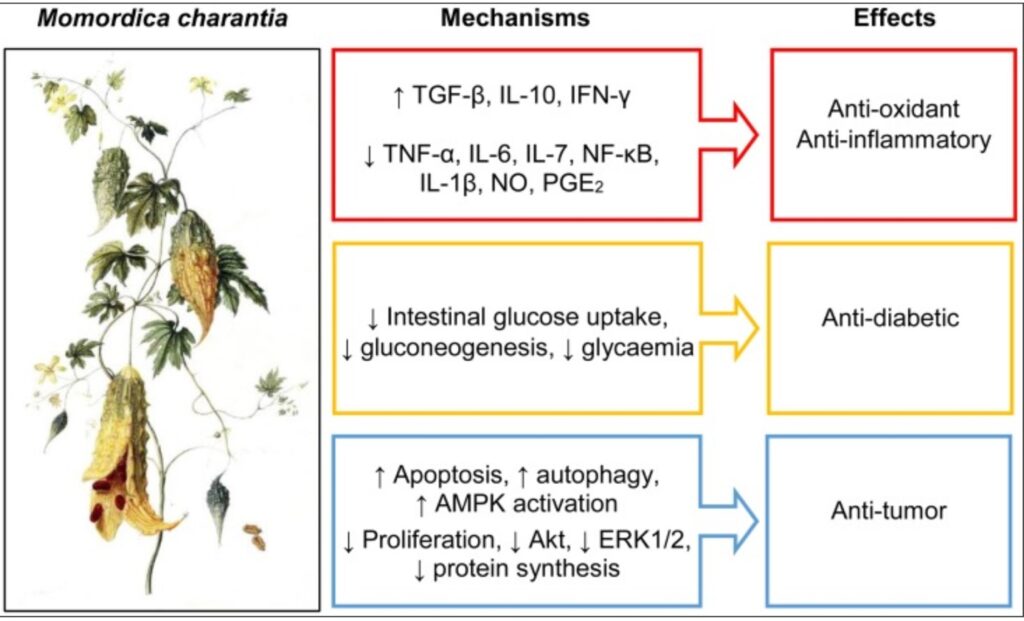
Bitter Melon Juice Modulates Glucose Metabolism and Lactate Efflux in Tumors in its Efficacy Against Pancreatic Cancer
Positron Emission Tomography ([18FDG]-PET) and Magnetic Resonance Imaging (MRI) on PANC1 tumor-bearing animals reiterated the in vitro results, with Bitter Melon Juice (BMJ) associated significant changes in tumor volumes, tumor cellularity and glucose uptake. Additional studies in BMJ treated pancreatic cells and xenografts displayed a strong decrease in the expression of glucose and lactate transporters GLUT1 and MCT4, respectively, supporting their role in metabolic changes by BMJ.[45]
Combinatorial Treatment with Natural Compounds in Prostate Cancer Inhibits Prostate Tumor Growth and Leads to Key Modulations of Cancer Cell Metabolism
A number of dietary phytochemicals, including curcumin (CUR), ursolic acid (UA), epigallocatechin-3-gallate (EGCG), resveratrol (RES), sulforaphane and 6-shogaol have shown potential chemopreventive effects in vitro and in vivo in animal models or in clinical studies on several cancers.
The effects of the most promising compounds (ursolic acid, curcumin, and resveratrol) were tested in vivo in a murine allograft model of prostate cancer as individual and combination treatments. All of the natural compound combinations resulted in synergistic effects on tumor volume and weight. A number of metabolic pathways were affected by the synergistic combinatorial treatments and allowed discrimination of those that were unequivocally driven by the individual compounds. Research indicates that a combination of ursolic acid with either curcumin or resveratrol leads to a blockade of glutamine uptake by the cancer cells, possibly contributing to the efficacy of these combinations in hindering prostate cancer growth.[46]
Additionally, Flavokawain A, a major chalcone in kava kava extract, has exhibited anti-proliferative and apoptotic effects in prostate cancer. Flavokawain A treatment regulates the glutamine metabolism pathway in prostate cancer cells by reducing intracellular glutamine, glutamic and proline uptake.[47]
Combinatorial Effect of Curcumin with Other Polyphenols and Anti-Cancer Drugs
Primary prostate cancer cells in B6C3F1/J mice when treated with the combination of curcumin and resveratrol increased the bioavailability by decreasing the tumor growth and inhibition of epithelial cell proliferation in contrast to curcumin administered alone. The combination of curcumin and resveratrol reduced prostate cancer by controlling the mean glucose uptake and decreasing the tumor weight of the mice.[48]
Cancer Cell Metabolic Reprograming Using Botanical Medicine
Reprogramming of cellular energy metabolism is widely accepted to be a cancer hallmark. Cancer cells have higher glucose needs and thus display a higher sensitivity to glucose deprivation-induced death than normal cells. So, inhibitors of glucose uptake are potential therapeutic targets in cancer.[49] Resveratrol, for example, suppresses cancer cell glucose uptake and glycolytic metabolism in a manner that depends on the capacity of resveratrol to inhibit intracellular ROS (reactive oxygen species), which downregulates HIF-1α accumulation.[50]
The flavonoids quercetin and the phenolic compound in green tea, epigallocatechin-3-gallate (EGCG) (26 min) concentration-dependently inhibited glucose uptake by breast cancer MCF-7 cells. EGCG also inhibited Lactate dehydrogenase (LDH)-A activity and caused cell apoptosis. LDH-A is regulated by HIF-1a and EGCG caused dissociation of Hsp90 from HIF-1a and subsequent HIF-1a degradation. EGCG also inhibited breast cancer cell growth in vivo, HIF-1a- and LDH-A- expression and triggered apoptosis without significant toxic side effects. EGCG can be considered as a pharmacologically effective compound to inhibit HIF-1a and LDH-A in cancer cells. [51]
Modern research validates the understanding of cancer as a metabolic disease, underscoring the need for a comprehensive, personalized approach.[52] Protocols therefore, must be designed to correct multiple underlying issues by altering cancer cell metabolism and reprogramming cell behavior with botanical, nutritional, dietary and lifestyle medicine, while simultaneously using a psycho-spiritual approach to reduce chronic stress. The fundamental objective of my approach (Mederi Care) is to build robustness and improve auto-regulation and auto-organization at the molecular, cellular and organ system levels.
[1] Bode, A. M. & Dong, Z. Cancer prevention research—then and now. Nat. Rev. Cancer 9, 508–516 (2009).
Lee, K. W., Bode, A. M. & Dong, Z. Molecular targets of phytochemicals for cancer prevention. Nat. Rev. Cancer 11, 211–218 (2011).
63 Lodi A, Saha A, Lu X, et al. Erratum: Combinatorial treatment with natural compounds in prostate cancer inhibits prostate tumor growth and leads to key modulations of cancer cell metabolism. NPJ Precis Oncol. 2017;1(1):30. Published 2017 Sep 4. doi:10.1038/s41698-017-0027-9
64 Muresanu C, Somasundaram SG, Vissarionov SV, et al. Updated Understanding of Cancer as a Metabolic and Telomere-Driven Disease, and Proposal for Complex Personalized Treatment, a Hypothesis. Int J Mol Sci. 2020;21(18):E6521. Published 2020 Sep 7. doi:10.3390/ijms21186521
[4] Singh, B., Singh, H. B., Singh, A., Naqvi, A. H. & Singh, B. R. Dietary phytochemicals alter epigenetic events and signaling pathways for inhibition of metastasis cascade. Cancer Metastasis Rev. 33, 41–85 (2014).
[5] Surh, Y. J. Cancer chemoprevention with dietary phytochemicals. Nat. Rev. Cancer 3, 768–780 (2003)
[6] Guerra AR, Duarte MF, Duarte IF Targeting Tumor Metabolism with Plant-Derived Natural Products: Emerging Trends in Cancer Therapy, J Agric Food Chem. 2018 Oct 17;66(41):10663-10685. doi: 10.1021/acs.jafc.8b04104.
[7] Elf SE, Chen J. Targeting glucose metabolism in patients with cancer. Cancer. 2014 Mar 15; 120(6):774-80.
[8] Zhu WW, Lu M, Wang XY, Zhou X, Gao C, Qin LX. The fuel and engine: The roles of reprogrammed metabolism in metastasis of primary liver cancer. Genes Dis. 2020;7(3):299-307. Published 2020 Feb 7. doi:10.1016/j.gendis.2020.01.016
[9] Metabolic reprogramming and cancer progression, randon Faubert, Ashley Solmonson, Ralph J. DeBerardinis, Science 10 Apr 2020:, Vol. 368, Issue 6487, eaaw5473, DOI: 10.1126/science.aaw5473
[10] Vasquez and Borniger, Neuroendocrine and Behavioral Consequences of Hyperglycemia in Cancer, May 2020, 161(5):1–10, ORCiD number: 0000-0002-5419-0271 (J. C. Borniger).
[11] P Freund, M A Kerenyi, M Hager, T Wagner, B Wingelhofer, H T T Pham, M Elabd, X Han, P Valent, F Gouilleux, V Sexl, O H Krämer, B Groner, R Moriggl. O-GlcNAcylation of STAT5 controls tyrosine phosphorylation and oncogenic transcription in STAT5-dependent malignancies. Leukemia, 2017; DOI: 10.1038/leu.2017.4
[12] Wang, L., & Wang, L. (2020). Identification of an energy metabolism‑related gene signature in ovarian cancer prognosis. Oncology Reports, 43, 1755-1770. https://doi.org/10.3892/or.2020.7548
[13] Muntean DM, Sturza A, Pavel IZ, Duicu OM. Modulation of Cancer Metabolism by Phytochemicals – A Brief Overview, Anticancer Agents Med Chem. 2018;18(5):684-692. doi: 10.2174/1871520617666171114102218.
[14] Yang, L. et al. Metabolic shifts toward glutamine regulate tumor growth, invasion and bioenergetics in ovarian cancer. Molecular systems biology 10, 728, doi:10.1002/msb.20134892 (2014).
[15] Caneba, C. A., Bellance, N., Yang, L., Pabst, L. & Nagrath, D. Pyruvate uptake is increased in highly invasive ovarian cancer cells under anoikis conditions for anaplerosis, mitochondrial function, and migration. American journal of physiology. Endocrinology and metabolism 303, E1036–1052, doi:10.1152/ajpendo.00151.2012 (2012).
[16] Pasto, A. et al. Cancer stem cells from epithelial ovarian cancer patients privilege oxidative phosphorylation, and resist glucose deprivation. Oncotarget 5, 4305–4319, doi:10.18632/oncotarget.2010 (2014).
[17] Dang, C. V., Le, A. & Gao, P. MYC-induced cancer cell energy metabolism and therapeutic opportunities. Clinical cancer research: an official journal of the American Association for Cancer Research 15, 6479–6483, doi:10.1158/1078-0432.CCR-09-0889 (2009).
[18] Feron, O. Pyruvate into lactate and back: from the Warburg effect to symbiotic energy fuel exchange in cancer cells. Radiotherapy and oncology: journal of the European Society for Therapeutic Radiology and Oncology 92, 329–333, doi:10.1016/j.radonc.2009.06.025, (2009).
[19] Csibi, A. et al. The mTORC1 pathway stimulates glutamine metabolism and cell proliferation by repressing SIRT4. Cell 153, 840–854, doi:10.1016/j.cell.2013.04.023 (2013).
[20] Sajad Dar1 , Jasdeep Chhina1 , Ismail Mert2 , Dhananjay Chitale3 , Thomas Buekers1 , Hareena Kaur1 , Shailendra Giri4 , Adnan Munkarah1 & Ramandeep Rattan, Bioenergetic Adaptations in Chemoresistant Ovarian Cancer Cells, SCIENTIFIC REPORTS | 7: 8760 | DOI:10.1038/s41598-017-09206-0
[21] Park-Min KH. Metabolic reprogramming in osteoclasts, Semin Immunopathol. 2019 Sep;41(5):565-572. doi: 10.1007/s00281-019-00757-0.
[22] WARBURG O., On the origin of cancer cells. Science. 1956 Feb 24; 123(3191):309-14.
[23] Magaway C, Kim E, Jacinto E,Targeting mTOR and Metabolism in Cancer: Lessons and Innovations, Cells. 2019 Dec 6;8(12). pii: E1584. doi: 10.3390/cells8121584.
[24] Magaway C, Kim E, Jacinto E,Targeting mTOR and Metabolism in Cancer: Lessons and Innovations, Cells. 2019 Dec 6;8(12). pii: E1584. doi: 10.3390/cells8121584.
[25] Chen, J. Xie, Z. Jiang, B. Wang, Y. Wang, and X. Hu, “Shikonin and its analogs inhibit cancer cell glycolysis by targeting tumor pyruvate kinase-M2,” Oncogene, vol. 30, no. 42, pp. 4297–4306, 2011
[26] Glenister A, Simone MI, Hambley TW. A Warburg effect targeting vector designed to increase the uptake of compounds by cancer cells demonstrates glucose and hypoxia dependent uptake. PLoS One. 2019 Jul 15;14(7):e0217712. doi: 10.1371/journal.pone.0217712. PMID: 31306426; PMCID: PMC6629077.
[27] Meziou, S., Ringuette Goulet, C., Hovington, H. et al. GLUT1 expression in high-risk prostate cancer: correlation with 18F-FDG-PET/CT and clinical outcome. Prostate Cancer Prostatic Dis 23, 441–448 (2020). https://doi.org/10.1038/s41391-020-0202-x
[28] Mimeault, M., Batra, SK., Hypoxia-inducing factors as master regulators of stemness properties and altered metabolism of cancer- and metastasis initiating cells, J Cell Mol Med . 2013 January ; 17(1): 30–54. doi:10.1111/jcmm.12004.
[29] Lee DH, Cheul Oh S, Giles AJ, Jung J, Gilbert MR, Park DM. Cardiac glycosides suppress the maintenance of stemness and malignancy via inhibiting HIF-1α in human glioma stem cells. Oncotarget. 2017;8(25):40233-40245. doi:10.18632/oncotarget.16714
[30] David C. Montrose, Lorenzo Galluzzi, Drugging cancer metabolism: Expectations vs. reality, Int Rev Cell Mol Biol. 2019 ; 347: 1–26. doi:10.1016/bs.ircmb.2019.07.007.
[31] Hay, N. Reprogramming glucose metabolism in cancer: can it be exploited for cancer therapy?. Nat Rev Cancer 16, 635–649 (2016). https://doi.org/10.1038/nrc.2016.77
[32] Lyons CL, Roche HM. Nutritional Modulation of AMPK-Impact upon Metabolic-Inflammation. Int J Mol Sci. 2018 Oct 9;19(10):3092. doi: 10.3390/ijms19103092. PMID: 30304866; PMCID: PMC6213547.
[33] Ubaldo E. Martinez-Outschoorn, Zhao Lin, Diana Whitaker-Menezes, Anthony Howell, Federica Sotgia, Michael P. Lisanti, Ketone body utilization drives tumor growth and metastasis, Cell Cycle 11:21, 3964–3971; November 1, 2012
[34] Kang HB, Fan J, Lin R, et al. Metabolic Rewiring by Oncogenic BRAF V600E Links Ketogenesis Pathway to BRAF-MEK1 Signaling. Mol Cell. 2015;59(3):345-358. doi:10.1016/j.molcel.2015.05.037
[35] Ahmad Salimi a , Mehryar Habibi Roudkenar b , Leila Sadeghi c , Alireza Mohseni b ,
Enayatollah Seydi a , Nahal Pirahmadi a,d , Jalal Pourahmad, Ellagic acid, a polyphenolic compound, selectively induces ROS-mediated apoptosis in cancerous B-lymphocytes of CLL patients by directly targeting mitochondria, Redox Biology 6 (2015) 461–471.
[36] Muntean DM, Sturza A, Pavel IZ, Duicu OM. Modulation of Cancer Metabolism by Phytochemicals – A Brief Overview. Anticancer Agents Med Chem. 2018; 18(5):684-692.
[37] Wang L, Li YM, Lei L, Liu Y, Wang X, Ma KY, Zhang C, Zhu H, Zhao Y, Chen ZY. Exp Gerontol. 2016 Sep; 82:95-103. Epub 2016 Jun 18.
[38] Li WL1, Yu HY1, Zhang XJ1, Ke M1, Hong T1. Purple sweet potato anthocyanin exerts antitumor effect in bladder cancer, Oncol Rep. 2018 Jul;40(1):73-82. doi: 10.3892/or.2018.6421
[39] Guerra AR, Duarte MF, Duarte IF Targeting Tumor Metabolism with Plant-Derived Natural Products: Emerging Trends in Cancer Therapy, J Agric Food Chem. 2018 Oct 17;66(41):10663-10685. doi: 10.1021/acs.jafc.8b04104.
[40] Zhangfeng Zhong,William W. Qiang, Wen Tan, 3 Haotian Zhang,Shengpeng Wang, Chunming Wang, Wenan Qiang,Yitao Wang, Chinese Herbs Interfering with Cancer Reprogramming Metabolism, Evid Based Complement Alternat Med. 2016; 2016: 9282813.
[41] Hongxiang Hui, George Tang, Vay Liang W Go, Hypoglycemic herbs and their action mechanisms, Chin Med. 2009; 4: 11. Published online 2009 Jun 12. doi: 10.1186/1749-8546-4-11
[42] Barbosa AM, Martel F. Targeting Glucose Transporters for Breast Cancer Therapy: The Effect of Natural and Synthetic Compounds. Cancers (Basel). 2020;12(1):154. Published 2020 Jan 8. doi:10.3390/cancers12010154
[43] Sur S, Nakanishi H, Flaveny C, Ippolito JE, McHowat J, Ford DA, Ray RB. Inhibition of the key metabolic pathways, glycolysis and lipogenesis, of oral cancer by bitter melon extract. Cell Commun Signal. 2019 Oct 21;17(1):131. doi: 10.1186/s12964-019-0447-y. Erratum in: Cell Commun Signal. 2019 Nov 19;17(1):151
[44] Bortolotti M, Mercatelli D, Polito L. Momordica charantia, a Nutraceutical Approach for Inflammatory Related Diseases. Front Pharmacol. 2019 May 8;10:486. doi: 10.3389/fphar.2019.00486. eCollection 2019. Review.
[45] Dhar D, Raina K, Kant R, Wempe MF, Serkova NJ, Agarwal C, Agarwal R. Bitter melon juice-intake modulates glucose metabolism and lactate efflux in tumors in its efficacy against pancreatic cancer. Carcinogenesis. 2019 Jun 13. pii: bgz114. doi: 10.1093/carcin/bgz114
[46] Lodi, A., Saha, A., Lu, X. et al. Combinatorial treatment with natural compounds in prostate cancer inhibits prostate tumor growth and leads to key modulations of cancer cell metabolism. npj Precision Onc 1, 18 (2017). https://doi.org/10.1038/s41698-017-0024-z
[47] Wang K, Zhang W, Wang Z, et al. Flavokawain A inhibits prostate cancer cells by inducing cell cycle arrest and cell apoptosis and regulating the glutamine metabolism pathway. J Pharm Biomed Anal. 2020;186:113288. doi:10.1016/j.jpba.2020.113288
[48] Liposome encapsulation of curcumin and resveratrol in combination reduces prostate cancer incidence in PTEN knockout mice. Narayanan N.K., Nargi D., Randolph C., Narayanan B.A. Int. J. Cancer. 2009;125:1–8. doi: 10.1002/ijc.24336
[49] Barbosa AM, Martel F. Targeting Glucose Transporters for Breast Cancer Therapy: The Effect of Natural and Synthetic Compounds. Cancers (Basel). 2020 Jan 8;12(1):154. doi: 10.3390/cancers12010154. PMID: 31936350; PMCID: PMC7016663.
[50] Kyung-Ho Jung, Jin Hee Lee, Cung Hoa Thien Quach, Jin-Young Paik, Hyunhee Oh, Jin Won Park, Eun Jeong Lee, Seung-Hwan Moon and Kyung-Han Lee, Resveratrol Suppresses Cancer Cell Glucose Uptake by Targeting Reactive Oxygen Species–Mediated Hypoxia-Inducible Factor-1α Activation, Jornal of Nuclear Medicine December 2013, 54 (12) 2161-2167; DOI: https://doi.org/10.2967/jnumed.112.115436
[51] Moreira L, Araújo I, Costa T, Correia-Branco A, Faria A, Martel F, Keating E. Quercetin and epigallocatechin gallate inhibit glucose uptake and metabolism by breast cancer cells by an estrogen receptor-independent mechanism. Exp Cell Res. 2013 Jul 15; 319(12):1784-1795.
[52] Muresanu C, Somasundaram SG, Vissarionov SV, et al. Updated Understanding of Cancer as a Metabolic and Telomere-Driven Disease, and Proposal for Complex Personalized Treatment, a Hypothesis. Int J Mol Sci. 2020;21(18):E6521. Published 2020 Sep 7. doi:10.3390/ijms21186521
Another excellent article. And yes. I remember studying macrobiotics in NYC at The Center in Soho (now closed) where it was taught that to heal cancer, one must avoid naturally sweet fruits and many vegetables. Purple sweet potatoes, with all their nutritional goodness, is a stunning reminder that this theory is deeply misunderstood. So thank you, again, Donnie for enlightening your readers.
Thanks for the comprehensive article on the metabolic approach to cancer disease. Your last paragraph summarized in laymen terms the essence of the research you shared. It would take me some time to digest all the scientific references and acronyms used in this blog post, but being a patient undergoing immunotherapy, I am familiar with some the terminology. I had not heard of the book, ‘My Grape Cure’. Believable considering all the ingredients in a purple grape.